Mainstream Electronic Product Battery Cell Product Series Parameters
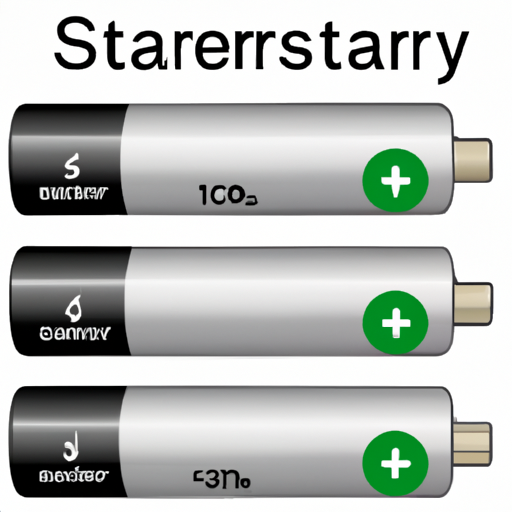
I. Introduction
In the modern world, battery cells are the lifeblood of electronic products, powering everything from smartphones and laptops to electric vehicles and renewable energy systems. As technology continues to advance, understanding the parameters of these battery cells becomes increasingly important for consumers, manufacturers, and researchers alike. This article aims to provide a comprehensive overview of the various types of battery cells, their key parameters, safety considerations, environmental impacts, and future trends in battery technology.
II. Types of Battery Cells
A. Lithium-ion (Li-ion) Batteries
Lithium-ion batteries are the most widely used battery technology in consumer electronics today. They are known for their high energy density, lightweight design, and ability to recharge quickly. Common applications include smartphones, laptops, tablets, and electric vehicles. The characteristics of Li-ion batteries include a nominal voltage of around 3.7 volts and a cycle life of approximately 500 to 1,500 charge cycles, depending on the specific chemistry used.
B. Nickel-Metal Hydride (NiMH) Batteries
NiMH batteries are another popular choice, particularly in hybrid vehicles and rechargeable household products. They offer a higher capacity than traditional nickel-cadmium batteries and are less toxic. NiMH batteries typically have a nominal voltage of 1.2 volts and can provide a cycle life of around 500 to 1,000 cycles. Their applications range from power tools to digital cameras.
C. Lead-Acid Batteries
Lead-acid batteries have been around for over a century and are primarily used in automotive applications, such as starting, lighting, and ignition systems. They are known for their robustness and low cost but have a lower energy density compared to Li-ion and NiMH batteries. Lead-acid batteries typically have a nominal voltage of 2 volts per cell and can last for about 500 cycles.
D. Emerging Technologies
The battery landscape is evolving, with emerging technologies like solid-state batteries and lithium-sulfur batteries showing promise for the future. Solid-state batteries use a solid electrolyte instead of a liquid one, potentially offering higher energy densities and improved safety. Lithium-sulfur batteries, on the other hand, have the potential for significantly higher energy densities than traditional lithium-ion batteries, making them an exciting area of research.
III. Key Parameters of Battery Cells
Understanding the key parameters of battery cells is crucial for evaluating their performance and suitability for specific applications.
A. Capacity
Capacity, measured in milliampere-hours (mAh) or ampere-hours (Ah), indicates the amount of energy a battery can store. A higher capacity means longer usage times for devices. For instance, a smartphone battery with a capacity of 3,000 mAh can typically last a full day under normal usage conditions.
B. Voltage
Voltage is a critical parameter that affects device compatibility. The nominal voltage is the average voltage a battery provides during discharge, while the maximum voltage is the peak voltage when fully charged. For example, a Li-ion battery has a nominal voltage of 3.7 volts but can reach up to 4.2 volts when fully charged. Understanding these values is essential for ensuring that batteries are compatible with the devices they power.
C. Energy Density
Energy density, expressed in watt-hours per liter (Wh/L) or watt-hours per kilogram (Wh/kg), measures how much energy a battery can store relative to its size or weight. Higher energy density means lighter and more compact batteries, which is particularly important for portable electronics. Li-ion batteries typically have an energy density of around 150-250 Wh/kg, while NiMH batteries range from 60-120 Wh/kg.
D. Power Density
Power density refers to the amount of power a battery can deliver relative to its size or weight, measured in watts per liter (W/L) or watts per kilogram (W/kg). Applications requiring high power output, such as electric vehicles and power tools, benefit from batteries with high power density. Li-ion batteries excel in this area, making them suitable for high-performance applications.
E. Cycle Life
Cycle life indicates how many complete charge and discharge cycles a battery can undergo before its capacity significantly degrades. This parameter is crucial for consumers looking for longevity in their devices. Factors affecting cycle life include temperature, charge/discharge rates, and the depth of discharge. Li-ion batteries typically offer a cycle life of 500 to 1,500 cycles, while NiMH batteries may last around 500 to 1,000 cycles.
F. Charge/Discharge Rates
The charge/discharge rate, often expressed as a C-rate, indicates how quickly a battery can be charged or discharged relative to its capacity. For example, a 1C rate means the battery can be fully charged or discharged in one hour. Higher C-rates are essential for applications requiring rapid energy delivery, such as electric vehicles during acceleration.
G. Temperature Range
The operating temperature range of a battery is critical for performance and safety. Most batteries have specific temperature limits within which they operate optimally. For instance, Li-ion batteries typically function well between -20°C and 60°C. Extreme temperatures can affect performance, reduce cycle life, and pose safety risks, making it essential to consider temperature when designing battery-powered devices.
IV. Safety Considerations
Safety is a paramount concern when it comes to battery cells. Common safety issues include thermal runaway, short circuits, and overcharging, which can lead to fires or explosions. To mitigate these risks, battery management systems (BMS) are employed to monitor and control battery performance, ensuring safe operation. Additionally, regulatory standards and certifications, such as UL and CE, help ensure that batteries meet safety requirements.
V. Environmental Impact
The environmental impact of battery cells is an increasingly important topic. Lifecycle analysis of battery cells considers the environmental effects from raw material extraction to manufacturing, usage, and disposal. Recycling and disposal considerations are critical, as improper disposal can lead to environmental contamination. Innovations in sustainable battery technologies, such as the development of biodegradable batteries and improved recycling methods, are essential for reducing the ecological footprint of battery production and usage.
VI. Future Trends in Battery Technology
The future of battery technology is promising, with several trends shaping the landscape. Advancements in battery chemistry, such as the development of solid-state and lithium-sulfur batteries, could lead to higher energy densities and improved safety. Additionally, the integration of batteries with renewable energy sources, such as solar and wind, is crucial for creating sustainable energy systems. The role of artificial intelligence in battery management is also gaining traction, with AI algorithms optimizing charging and discharging processes to extend battery life and enhance performance.
VII. Conclusion
In conclusion, understanding the parameters of battery cells is essential for consumers and manufacturers alike. As technology continues to evolve, the importance of battery specifications in purchasing decisions cannot be overstated. With advancements in battery technology on the horizon, the future looks bright for mainstream electronics, promising longer-lasting, safer, and more efficient battery solutions.
VIII. References
1. N. J. G. et al., "A Review of Lithium-Ion Battery Safety Concerns: The Issues, Strategies, and Solutions," *Journal of Power Sources*, vol. 400, pp. 1-15, 2018.
2. D. Linden and T. B. Reddy, *Handbook of Batteries*, 4th ed. New York: McGraw-Hill, 2011.
3. "Battery Recycling: A Guide for Consumers," *Environmental Protection Agency*, 2020.
4. M. Winter and J. O. Besenhard, "Electrochemical Lithium Intercalation: A Review," *Journal of Power Sources*, vol. 68, pp. 1-9, 1997.
5. "The Future of Battery Technology," *International Energy Agency*, 2021.
This blog post provides a detailed overview of the parameters of battery cells used in mainstream electronic products, covering various types, key specifications, safety, environmental impact, and future trends. Understanding these aspects is crucial for making informed decisions in an increasingly battery-dependent world.
Mainstream Electronic Product Battery Cell Product Series Parameters
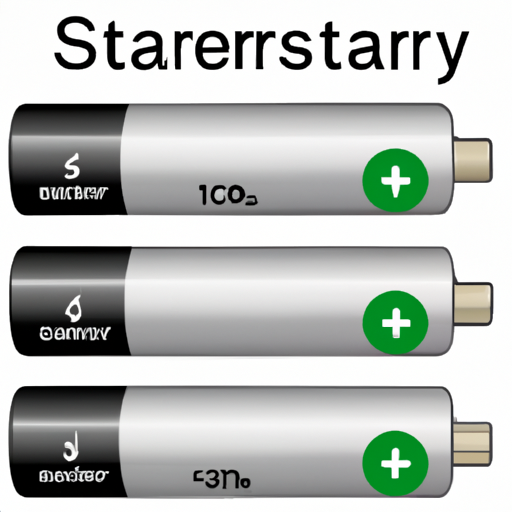
I. Introduction
In the modern world, battery cells are the lifeblood of electronic products, powering everything from smartphones and laptops to electric vehicles and renewable energy systems. As technology continues to advance, understanding the parameters of these battery cells becomes increasingly important for consumers, manufacturers, and researchers alike. This article aims to provide a comprehensive overview of the various types of battery cells, their key parameters, safety considerations, environmental impacts, and future trends in battery technology.
II. Types of Battery Cells
A. Lithium-ion (Li-ion) Batteries
Lithium-ion batteries are the most widely used battery technology in consumer electronics today. They are known for their high energy density, lightweight design, and ability to recharge quickly. Common applications include smartphones, laptops, tablets, and electric vehicles. The characteristics of Li-ion batteries include a nominal voltage of around 3.7 volts and a cycle life of approximately 500 to 1,500 charge cycles, depending on the specific chemistry used.
B. Nickel-Metal Hydride (NiMH) Batteries
NiMH batteries are another popular choice, particularly in hybrid vehicles and rechargeable household products. They offer a higher capacity than traditional nickel-cadmium batteries and are less toxic. NiMH batteries typically have a nominal voltage of 1.2 volts and can provide a cycle life of around 500 to 1,000 cycles. Their applications range from power tools to digital cameras.
C. Lead-Acid Batteries
Lead-acid batteries have been around for over a century and are primarily used in automotive applications, such as starting, lighting, and ignition systems. They are known for their robustness and low cost but have a lower energy density compared to Li-ion and NiMH batteries. Lead-acid batteries typically have a nominal voltage of 2 volts per cell and can last for about 500 cycles.
D. Emerging Technologies
The battery landscape is evolving, with emerging technologies like solid-state batteries and lithium-sulfur batteries showing promise for the future. Solid-state batteries use a solid electrolyte instead of a liquid one, potentially offering higher energy densities and improved safety. Lithium-sulfur batteries, on the other hand, have the potential for significantly higher energy densities than traditional lithium-ion batteries, making them an exciting area of research.
III. Key Parameters of Battery Cells
Understanding the key parameters of battery cells is crucial for evaluating their performance and suitability for specific applications.
A. Capacity
Capacity, measured in milliampere-hours (mAh) or ampere-hours (Ah), indicates the amount of energy a battery can store. A higher capacity means longer usage times for devices. For instance, a smartphone battery with a capacity of 3,000 mAh can typically last a full day under normal usage conditions.
B. Voltage
Voltage is a critical parameter that affects device compatibility. The nominal voltage is the average voltage a battery provides during discharge, while the maximum voltage is the peak voltage when fully charged. For example, a Li-ion battery has a nominal voltage of 3.7 volts but can reach up to 4.2 volts when fully charged. Understanding these values is essential for ensuring that batteries are compatible with the devices they power.
C. Energy Density
Energy density, expressed in watt-hours per liter (Wh/L) or watt-hours per kilogram (Wh/kg), measures how much energy a battery can store relative to its size or weight. Higher energy density means lighter and more compact batteries, which is particularly important for portable electronics. Li-ion batteries typically have an energy density of around 150-250 Wh/kg, while NiMH batteries range from 60-120 Wh/kg.
D. Power Density
Power density refers to the amount of power a battery can deliver relative to its size or weight, measured in watts per liter (W/L) or watts per kilogram (W/kg). Applications requiring high power output, such as electric vehicles and power tools, benefit from batteries with high power density. Li-ion batteries excel in this area, making them suitable for high-performance applications.
E. Cycle Life
Cycle life indicates how many complete charge and discharge cycles a battery can undergo before its capacity significantly degrades. This parameter is crucial for consumers looking for longevity in their devices. Factors affecting cycle life include temperature, charge/discharge rates, and the depth of discharge. Li-ion batteries typically offer a cycle life of 500 to 1,500 cycles, while NiMH batteries may last around 500 to 1,000 cycles.
F. Charge/Discharge Rates
The charge/discharge rate, often expressed as a C-rate, indicates how quickly a battery can be charged or discharged relative to its capacity. For example, a 1C rate means the battery can be fully charged or discharged in one hour. Higher C-rates are essential for applications requiring rapid energy delivery, such as electric vehicles during acceleration.
G. Temperature Range
The operating temperature range of a battery is critical for performance and safety. Most batteries have specific temperature limits within which they operate optimally. For instance, Li-ion batteries typically function well between -20°C and 60°C. Extreme temperatures can affect performance, reduce cycle life, and pose safety risks, making it essential to consider temperature when designing battery-powered devices.
IV. Safety Considerations
Safety is a paramount concern when it comes to battery cells. Common safety issues include thermal runaway, short circuits, and overcharging, which can lead to fires or explosions. To mitigate these risks, battery management systems (BMS) are employed to monitor and control battery performance, ensuring safe operation. Additionally, regulatory standards and certifications, such as UL and CE, help ensure that batteries meet safety requirements.
V. Environmental Impact
The environmental impact of battery cells is an increasingly important topic. Lifecycle analysis of battery cells considers the environmental effects from raw material extraction to manufacturing, usage, and disposal. Recycling and disposal considerations are critical, as improper disposal can lead to environmental contamination. Innovations in sustainable battery technologies, such as the development of biodegradable batteries and improved recycling methods, are essential for reducing the ecological footprint of battery production and usage.
VI. Future Trends in Battery Technology
The future of battery technology is promising, with several trends shaping the landscape. Advancements in battery chemistry, such as the development of solid-state and lithium-sulfur batteries, could lead to higher energy densities and improved safety. Additionally, the integration of batteries with renewable energy sources, such as solar and wind, is crucial for creating sustainable energy systems. The role of artificial intelligence in battery management is also gaining traction, with AI algorithms optimizing charging and discharging processes to extend battery life and enhance performance.
VII. Conclusion
In conclusion, understanding the parameters of battery cells is essential for consumers and manufacturers alike. As technology continues to evolve, the importance of battery specifications in purchasing decisions cannot be overstated. With advancements in battery technology on the horizon, the future looks bright for mainstream electronics, promising longer-lasting, safer, and more efficient battery solutions.
VIII. References
1. N. J. G. et al., "A Review of Lithium-Ion Battery Safety Concerns: The Issues, Strategies, and Solutions," *Journal of Power Sources*, vol. 400, pp. 1-15, 2018.
2. D. Linden and T. B. Reddy, *Handbook of Batteries*, 4th ed. New York: McGraw-Hill, 2011.
3. "Battery Recycling: A Guide for Consumers," *Environmental Protection Agency*, 2020.
4. M. Winter and J. O. Besenhard, "Electrochemical Lithium Intercalation: A Review," *Journal of Power Sources*, vol. 68, pp. 1-9, 1997.
5. "The Future of Battery Technology," *International Energy Agency*, 2021.
This blog post provides a detailed overview of the parameters of battery cells used in mainstream electronic products, covering various types, key specifications, safety, environmental impact, and future trends. Understanding these aspects is crucial for making informed decisions in an increasingly battery-dependent world.